New Insights into Lipid Binding and Membrane Protein Stabilization
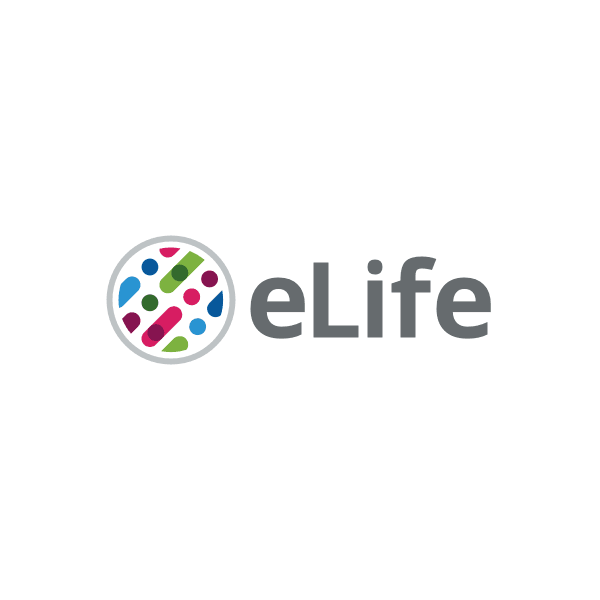
In a groundbreaking study, researchers have systematically examined the requirements necessary for the binding and stabilization of membrane proteins by a lipid-like molecule known as CDL (Cation-Dependent Lipid). This work utilized the scaffold protein ROCKET as a model system to shed light on the interactions between membrane proteins and lipids, which are crucial for various cellular functions.
The study reveals that CDL binding sites exhibit surprisingly few sequence requirements. This conclusion stems from the observation that ROCKET contains several interaction sites formed mainly through the statistical distribution of aromatic and charged amino acids located at the membrane interface. The interactions at these sites exhibit distinct characteristics, including electrostatic attractions with negatively charged lipid headgroups and neighboring aromatic residues that help align the lipid acyl chains with the hydrophobic transmembrane domain of the protein. The researchers introduced specific mutations at these sites to assess their roles in CDL-mediated stabilization using native Mass Spectrometry (nMS).
Interestingly, the results showed that although CDL binding sites possess well-defined structural features, the mutations aimed at altering these features did not consistently yield predictable outcomes in terms of structural stability. This unexpected behavior indicates that multiple additional factors influence the ability of these sites to contribute to lipid-mediated stabilization. Notably, mutations that reduce CDL binding at a high-affinity site can lead to a redistribution of interactions toward lower-affinity sites, which may exert a more significant effect on overall stability, as exemplified by the ROCKETAAXWA mutation. Conversely, mutations that diminish lipid binding at a low-affinity site can unexpectedly enhance lipid-mediated stabilization, as seen with the ROCKETR66A mutation. This suggests that lipid interactions are inherently dynamic and heterogeneous, allowing for the emergence of compensatory interactions that significantly influence the local environment of the protein.
Despite the complexities involved, the different CDL binding sites within ROCKET illustrate that commonly observed structural featuressuch as the number of headgroup-interaction residues, local flexibility, and the involvement of several transmembrane helicesimpact lipid-mediated stabilization to varying extents. Building on the insights obtained from ROCKET, the researchers further explored E. coli proteins, identifying GlpG as a compelling case study to investigate the functional impact of CDL binding. Previous studies have indicated that the conformation of GlpG is influenced by the surrounding lipids and the geometry of the membrane. Furthermore, it has been suggested that CDL may play a role in modulating the proteolytic activity of GlpG.
The researchers discovered that CDL functions as an allosteric activator, promoting the cleavage of soluble substrates while inhibiting the processing of transmembrane substrates. This positions CDL as a critical regulator of GlpG activity. A similar CDL binding site was identified in the yeast ATP/ADP carrier, Aac2, characterized by the residues W70/R71/R151. This binding site shares structural hallmarks with the GlpG binding site, demonstrating that CDL binding connects the flexible N-terminal transmembrane helix to the protein core, thereby stabilizing the tertiary structure and enhancing transport activity.
Although the team could identify somewhat intuitive features of CDL interaction sites, they found that the relationship between lipid binding and protein stabilization is not straightforward. For example, a mutation that destabilizes the protein may also increase lipid-mediated stabilization, while another might not have the same effect. The researchers compared the ROCKETR66A and ROCKETA61P mutations to illustrate this complexity. Additionally, the ability to bind more lipids does not necessarily correlate with the formation of more stable complexes, as demonstrated by the comparison between ROCKET and ROCKETAAXWA.
These discrepancies are likely due to a twofold issue: First, the methodological constraints of the study mean that gas-phase stability does not easily correlate with stability in a condensed phase. In the case of CDL, increasing the number of molecular contacts generally leads to stabilizing effects in both phases. However, charge interactions are often stronger in the gas phase, while certain hydrophobic contacts may be lost. For instance, CDL sites typically contain aromatic residues close to the lipid ester bonds, which probably play a role in orienting the lipids, although this aspect has not yet been fully explored in ROCKET. Unlike charge interactions, these subtle contributions may be obscured during the transition to the gas phase, complicating the assessment of changes in stability or lipid occupancy.
Moreover, much of the nMS analysis was conducted using detergents, which can strip lipids from proteins, leading to fewer observed lipid binding events and lower occupancy rates compared to Coarse-Grained Molecular Dynamics (CG-MD) simulations. The collisional activation required to remove a detergent micelle or lipid vesicle can further eliminate lipids that bind primarily through hydrophobic interactions.
To mitigate the limitations associated with their mass spectrometry approach, the researchers directed their analysis towards CDL that binds via direct headgroup contacts, a method that is more reliably predicted through CG-MD and compatible with nMS analysis. This study underscores the intricacies of protein-lipid interactions. The ROCKET scaffold, by design, is an extremely stable model lacking functional activity. Hence, it does not fully encapsulate the dynamic nature typical of most membrane proteins, which are often subject to lipid-mediated regulation.
The pivotal conclusion of this research is that simply replicating the architecture of a binding site is just the initial step toward understanding the principles governing lipid regulation. The effects of lipids on local dynamics hold significant importance in shaping how membrane proteins function. Consequently, integrating design methodologies based not only on static structures but also on dynamic models is essential for developing membrane proteins with specific functions.
In conclusion, this protein design-driven study provides important insights into how CDL regulates membrane protein stability. The findings illuminate critical features of proteins that are challenging to predict or design from scratch. Further integration of MD simulations with nMS and protein engineering may help address some of the challenges identified, including the influence of local protein flexibility. This research opens promising pathways for discovering novel CDL binding sites, enhancing our understanding of lipid-protein interactions, and potentially informing therapeutic strategies aimed at mitigating membrane protein dysfunction.