Unraveling Membrane Protein Stability: The Role of CDL Binding Sites
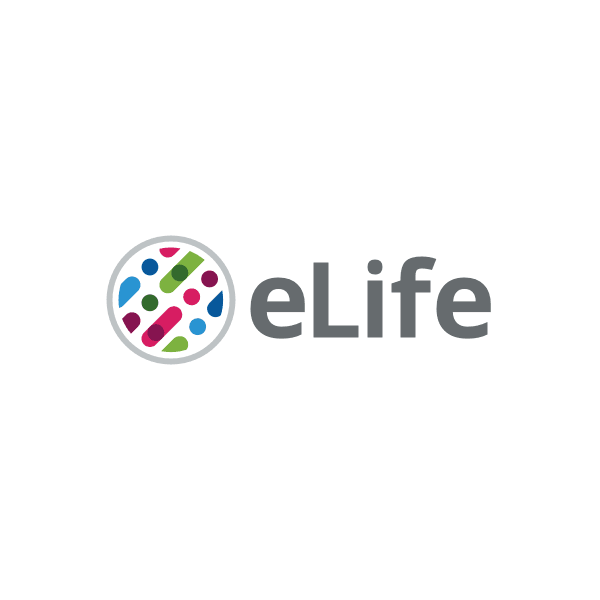
In a groundbreaking study, researchers have systematically explored the requirements for the binding and stabilization of membrane proteins via a specialized scaffold protein known as ROCKET. This investigation serves as a model system to gain insight into how CDL (charged lipid) binding sites operate. Remarkably, these CDL binding sites exhibit minimal sequence requirements, highlighted by the statistical distribution of aromatic and charged amino acids present at the membrane interface, as illustrated in Figure 1.
The binding sites of ROCKET reveal distinct and characteristic features. Notably, these include strong electrostatic interactions with negatively charged lipid headgroups, as well as flanking aromatic residues that help to orient the acyl chains with the hydrophobic transmembrane domain. By introducing targeted mutations at these sites, researchers have been able to probe the contributions these mutations make to CDL-mediated stabilization using native mass spectrometry (nMS). However, the findings were surprising: even though CDL binding sites share defined structural characteristics, mutations affecting these features dont always yield the anticipated structural changes. Instead, the stabilization potential is influenced by a multitude of additional factors.
For instance, mutations that diminish the affinity for a high-affinity CDL binding site can lead to a compensatory redistribution to lower-affinity sites, which may have a more significant impact on overall protein stability. This phenomenon was particularly noted in the case of the mutation ROCKETAAXWA. On the other hand, certain mutations that reduced lipid binding at a lower-affinity site resulted in enhanced lipid-mediated stabilization, such as observed with the ROCKETR66A mutation. Such findings indicate that lipid interactions are inherently dynamic and heterogeneous; thus, modifications at a defined CDL site can elicit compensatory interactions that affect the surrounding protein environment.
Despite these complexities, the multiple CDL binding sites identified in the ROCKET scaffold illustrate how commonly observed structural traitsincluding the number of residues that interact with headgroups, local protein flexibility, and the involvement of multiple transmembrane helicessignificantly influence lipid-mediated stabilization, albeit to varying degrees. Building on the insights gained from ROCKET, the research team expanded their focus to proteins found in E. coli, specifically investigating GlpG as an illustrative example of CDL's impact on protein function.
Previous studies have indicated that the conformation of GlpG is significantly influenced by the surrounding lipids and the geometry of the membrane (Yen et al., 2022; Vinothkumar, 2011). Furthermore, CDL has been posited to play a role in modulating GlpG's proteolytic activity (Urban and Wolfe, 2005). The researchers observed that CDL serves as an allosteric activator for the cleavage of soluble substrates, while simultaneously exerting an inhibitory effect on the processing of transmembrane substrates. This establishes CDL as a crucial regulator of GlpG activity.
In a parallel investigation, the researchers examined the CDL binding site (W70/R71/R151) within the yeast ATP/ADP carrier Aac2, which shares similar characteristics with the GlpG site, as depicted in Figure 4figure supplement 2. The binding of CDL to this site effectively connects the flexible N-terminal transmembrane helix to the protein core, thereby stabilizing the tertiary structure and enhancing transport activity. Through nMS analysis, it was established that the protein retains co-purified CDL (Senoo et al., 2024). These findings suggest that the features responsible for lipid stabilization in ROCKET are indicative of functionally significant lipid binding sites found in native membrane proteins.
Despite the ability to identify intuitive characteristics of CDL interaction sites, the relationship between lipid binding and stabilization remains elusive. Notably, one mutation that destabilizes the protein can simultaneously enhance lipid-mediated stabilization, while another mutation may not produce any such effect (as seen when comparing ROCKETR66A and ROCKETA61P). Moreover, an increased capacity for lipid binding does not necessarily equate to the formation of more stable complexes, as illustrated by the distinctions between ROCKET and ROCKETAAXWA.
These outcomes likely arise from two primary factors. Firstly, the methodological limitations of the research complicate the correlation between gas-phase stability and condensed-phase stability. In the case of CDL, an increased number of molecular contacts might indeed translate to stabilizing effects in both phases. However, charge interactions tend to be more pronounced in the gas phase, whereas certain hydrophobic contacts may diminish. For example, CDL sites contain aromatic residues (such as W or Y) situated adjacent to the ester bonds of lipids, which likely serve to orient lipids; however, the specific roles of these residues have not been thoroughly examined within ROCKET.
Unlike the charge interactions with lipid head groups, the subtle contributions of hydrophobic interactions are likely distorted when transitioning to the gas phase, complicating the interpretation of stability changes or lipid occupancy. The majority of nMS analyses are conducted using detergents, which can have delipidating effects, potentially leading to fewer lipid binding events and lower occupancy rates in nMS compared to coarse-grained molecular dynamics (CG-MD) simulations (Bolla et al., 2020). Furthermore, the collisional activation necessary to remove the detergent micelle or lipid vesicle strips away lipids that predominantly bind via hydrophobic interactions.
In light of these limitations, the researchers have intentionally focused on CDL bound through direct headgroup contacts, as these interactions can be accurately predicted using CG-MD and effectively analyzed through nMS. Secondly, their findings underscore the complexities inherent in protein-lipid interactions. The ROCKET scaffold is designed to be exceptionally stable and lacks functional properties, meaning it does not capture the dynamic nature of most membrane proteins. This dynamic nature is often linked to lipid-mediated regulation (Landreh et al., 2017). The critical takeaway from this study is that while emulating the architecture of a binding site serves as an initial step in uncovering lipid regulation principles, understanding the effects of lipids on local dynamics is vital for interpreting how they influence membrane protein functionality. Thus, integrated design approaches that consider not just static structures but also dynamic models are essential for developing membrane proteins with specific functions.
In conclusion, this research presents a robust, design-driven approach to deciphering the mechanisms by which CDL regulates membrane protein stability. The results illuminate crucial protein characteristics that pose challenges for prediction or de novo design. By further integrating molecular dynamics simulations and nMS with protein engineering, researchers can address the challenges identified, particularly the effects of local protein flexibility. This approach offers a promising pathway for discovering new CDL binding sites. Ultimately, these findings enhance our understanding of lipid-protein interactions and highlight potential avenues for designing membrane proteins with tailored stability and functionality, potentially paving the way for therapeutic strategies targeting membrane protein dysfunction.