New Insights into Midline Barriers and Left-Right Asymmetry in Embryonic Development
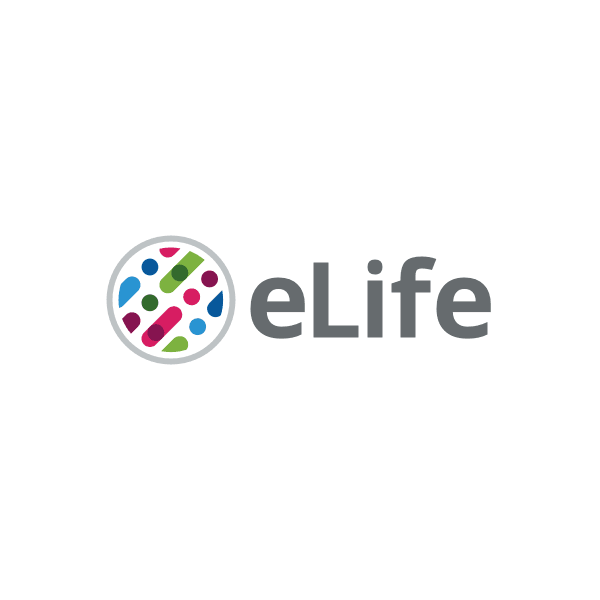
Understanding the establishment of the left and right body plan in early embryos is crucial for developmental biology, as this process significantly influences organ formation and overall body structure. Recent studies highlight the essential role of the Lefty1+ midline barrier in this context. While individual organs exhibit left-right (LR) asymmetries, the brain uniquely possesses an organ-specific midline barrier. This specialized barrier regulates the commissural axons of both the brain and spinal cord, employing localized guidance and repulsion cues that include critical signaling pathways such as FGFs, SLIT/ROBO, EFNB3, heparan sulfate proteoglycans, and the Rac-specific GTPase-activating protein -chimaerin (Cavalcante et al., 2002; Kullander et al., 2001; Brose et al., 1999; Kidd et al., 1998; Erskine et al., 2000; Neugebauer and Yost, 2014; Katori et al., 2017).
In contrast, the developing intestine requires a similar mechanism to maintain separation between left and right cell signals. However, it appears to achieve this through an atypical basement membrane located at the midline, which acts as a barrier to segregate cells originating from the right and left coelomic epithelia (Carmona et al., 2013). Remarkably, these cells do not intermingle at the midline, suggesting a highly organized structure (Figure 2). The ability of diffusible signals to cross this midline barrier is context-dependent; some morphogens, such as Nodal, can exert effects over distances exceeding 200 m (Mller and Schier, 2011). Given that the HH19 DM has a width of only about 150 m, it raises the question of whether the midline barrier is essential for preventing the mixing of these crucial signals.
Our initial hypothesis proposed that the DM requires a barrier at the midline to prevent the diffusion of various signals. Supporting this notion, we demonstrated that the midline effectively limits the diffusion of dextran from right to left, indicating it might also inhibit the movement of other endogenous diffusible signals. Notably, dextran, with a molecular weight of just 3 kDa, was unable to traverse the midline, suggesting that larger proteins, such as CXCL12 (10 kDa) and BMP4 (34 kDa), are also restricted from crossing this barrier. Previous findings had shown that even the small drug AMD3100 (502.78 Da) could not migrate across the midline (Mahadevan et al., 2014).
Further investigations employed a BODIPY-labeled version of AMD3100 (Poty et al., 2015). Under conditions where the basement membrane remained intact, BODIPY diffusion across the midline was severely restricted. Conversely, in the absence of the basement membrane, BODIPY diffusion occurred freely across the full width of the DM. While our experiments indicated that midline degradation could not be induced through Ntn4 overexpression, future studies may identify specific techniques to selectively dismantle this basement membrane, providing deeper insights into its role in gut laterality.
This research sheds light on the complex structure and function of basement membranes, which are vital for both embryonic and adult development. Typically, basement membranes exist as a single layer underpinning polarized epithelial or endothelial cells, such as those found in the intestines or surrounding blood vessels (Yurchenco, 2011). Mutations that disrupt the genes responsible for basement membrane components often lead to embryonic lethality or various postnatal health issues (Bader et al., 2005; Miner et al., 2004; Smyth et al., 1999; Gatseva et al., 2019; Pozzi et al., 2017; Yao, 2017). However, the specific role of basement membranes in establishing left-right asymmetry had not been previously documented.
The basement membrane identified in this study is unique due to its double membrane structure, prompting intriguing questions regarding its development. Our research indicated that the midline is not formed by mesenchymal cells of the DM (Figure 4), nor is the notochord sufficient for its synthesis (Figure 4). Instead, we suspect the endoderm plays a critical role. Electroporation of the endoderm with GFP did not result in GFP-positive cells in the DM at later developmental stages, suggesting that the midline does not derive from epithelial-mesenchymal transition (EMT) of basement membrane-containing endodermal cells (Figure 4FH). This finding is reinforced by the absence of significant cell death at the midline during the stages of interest, as demonstrated by TUNEL staining (data not shown).
Additionally, the midline does not form in the same manner as other double basement membranes, which are typically the result of two tissues meeting at their basal sides (Keeley and Sherwood, 2019; Pastor-Pareja, 2020). Classic examples include the kidney glomerulus, where epithelial podocytes and endothelial cells meet (Pastor-Pareja, 2020; Miner, 2012; Naylor et al., 2021), and the blood-brain barrier, formed by a combination of endothelial cells, pericytes, and astrocytes (Keeley and Sherwood, 2019; Daneman and Prat, 2015). In the specific case of the DM midline barrier, the apical surfaces of the endodermal cells face one another.
We hypothesize that the midline arises when the basement membrane is left behind as the endoderm descends ventrally during normal development, akin to a scar marking the former position of the endoderm (Figure 7). During early development, the notochord is situated within the endoderm, covered by a basement membrane that connects the two structures. Ultimately, these two structures become separated by a complete basement membrane, suggesting a strong interrelationship (Fausett et al., 2014; Jurand, 1974). Tissue forces generated by the medial migration of the aortae and coelomic epithelia may push the notochord and endoderm apart, leading to the residual basement membrane at the DM midline. Furthermore, the basement membranes of both the endoderm and midline exhibited resistance to disruption by NTN4, suggesting that the endoderm may be responsible for synthesizing both structures (Figure 7).
This study also suggests that the midline barrier's resistance to NTN4 disruption points to an unidentified factor or modification that stabilizes the laminin network against NTN4 binding. Understanding the mechanisms that uphold basement membranes against such disruptions could be crucial for future developmental studies and research concerning diseases like cancer.
While Lefty1 expression is absent at the midline when the basement membrane is strong, its role in the construction or function of this structure remains uncertain. Future research could investigate the effects of siRNA inhibition of Lefty1 expression during early stages, assessing the integrity of the midline structure at HH19. Yet, this approach may pose challenges in distinguishing between the direct influence of Lefty1 loss on future midline cells and the indirect effects stemming from the unrestricted diffusion of Nodal throughout the early lateral mesoderm due to Lefty deficiency. A more profound understanding of the relationship between these two aspects of the midline barrier is essential.
One distinctive feature of the midline barrier is its rapid disintegration. We propose that the loss of the DM midline barrier may arise partly from stretching of the basement membrane as the notochord and endoderm become increasingly separated due to the elongation of the DM (Figure 3J). Since the DM does not contribute basement membrane to the midline (Figure 4C) and the midline length expands swiftly (Figure 3J), it is plausible that the midline is pulled until it reaches its ultimate tensile strengthestimated between 0.5 and 3.8 MPa in other naturally occurring basement membranes (Jain et al., 2022)leading to its rupture. This indicates that midline breakdown may be a passive consequence of embryonic growth.
However, we also consider the possibility of an active breakdown mechanism for the midline. The turnover of stable basement membranes generally spans weeks (Trier et al., 1990; Decaris et al., 2014), but the midline barrier seems to disintegrate within just 12 to 24 hours. In other contexts, destruction of the basement membrane can occur over large areas, such as through the secretion of matrix metalloproteinases during cancer metastasis (Miyoshi et al., 2004; Miyoshi et al., 2005), or through localized actions by invadopodia on immune or cancer cells prior to metastasis (Sekiguchi and Yamada, 2018; Santiago-Medina et al., 2015). Such localized breakdown of basement membranes is evident in essential developmental processes, including the formation of the mouth in deuterostome embryos (Dickinson and Sive, 2006). The oral membrane, which isolates the digestive system from the exterior, specifically disintegrates to create an opening for the early mouth cavity (Dickinson and Sive, 2006). Similarly, localized breakdown of basement membranes is critical for inter-hemisphere communication in the cerebrum, where the corpus callosum must be disrupted to facilitate neuron crossing (Hakanen and Salminen, 2015; Gobius et al., 2016). It is noteworthy that in all these instances, the breakdown of the basement membrane occurs at specific sites, leaving adjacent membranes intact (Figure 3H). Collectively, these findings imply that the breakdown of the DM midline may not merely be a passive event; rather, it could be essential for subsequent gut development or vascular patterning, highlighting the need for further investigation to identify any matrix proteases specific to the midline of the DM.
Interestingly, the DM midline barrier may have implications that extend beyond the intestine. Signals generated from axial structures play a pivotal role in establishing LR asymmetry, yet pinpointing the actual midline structure or midline signal responsible has proven challenging. Structures such as the heart (Lohr et al., 1997; Chen et al., 1997), lungs (Arraf et al., 2016), and kidneys (James and Schultheiss, 2003) all depend on dorsal midline structures for proper development. Disruptions caused by the absence of midline structures like the notochord can lead to conditions like horseshoe kidney, where the kidneys remain near the midline and fuse at their posterior ends (Natsis et al., 2014). This phenomenon arises from the lack of Shh signaling from the notochord, yet the downstream midline barrier resulting from this Shh signaling is still enigmatic (Tripathi et al., 2010).
Moreover, the DM midline barrier may influence vascular patterning, particularly concerning aortic fusion. Initially, the aorta forms as two parallel tubes with an avascular zone in between (coinciding with the midline) (Garriock et al., 2010). These tubes progressively fuse, advancing from anterior to posterior until reaching the vitelline arteries (Figure 3figure supplement 3; Garriock et al., 2010). The timing of this fusion correlates with the fragmentation and disappearance of the midline, suggesting a close connection between these processes. The precise timing of fusion relies on a delicate balance among VEGF (Jadon et al., 2023), SHH (Vokes et al., 2004), and the anterior-to-posterior downregulation of BMP-inhibiting genes like Chordin and Noggin from the notochord (Garriock et al., 2010; Reese et al., 2004; Sato, 2013). The exact mechanism behind dorsal aorta fusion remains unknown, although existing evidence indicates that VEGF signaling may pull VE-cadherin away from its cell-cell junctions, which might be crucial for remodeling the aortic endothelium during fusion and could relate to the disruption of the DM midline barrier.
Midline structures are vital for the correct development of laterality. The notochord plays a significant role here, especially as a source of modulators for BMP and Hedgehog signaling. Nevertheless, this does not seem to encompass the entire picture, given that the actual midline barrier following notochord signaling has yet to be identified. It is plausible that the DM midline basement membrane is critical, either in separating left and right signals or perhaps in serving as a reservoir for signals (Pozzi et al., 2017) from the notochord, thereby acting as a buffer between the two sides. Additionally, the midline barrier might also influence the rheology of the DM. Microindentation analyses conducted at HH21 reveal that the condensed left DM exhibits significantly greater stiffness compared to the expanded right DM, suggesting that the precise regulation of this stiffness is crucial for proper gut tilting (Sanketi et al., 2022). The midline barrier likely contributes to the segregation of stiffness-influencing components on each side (i.e., covalently modified HA on the right [Sivakumar et al., 2018], N-cadherin on the left [Kurpios et al., 2008]), and may serve as a wall against which the right side can exert force, facilitating the movement of the gut tube toward the left. It is noteworthy that left and right mesenchymal cells do not cross the midline to the opposite side of the DM. Similarly, during the formation of gut arteries, a small subset of vascular endothelial cells migrate from right to left, closely adjacent to the dorsal tip of the endoderm, yet they do not traverse the midline (Mahadevan et al., 2014). When the midline basement membrane remains intact, N-cadherin expression is symmetrical across the DM, but becomes asymmetric and left-specific upon midline disintegration (Kurpios et al., 2008). This suggests a shift in the mechanisms of cellular segregation in the DMwhile the midline is preserved, the double basement membrane structure suffices to separate left and right cells. However, when the midline disappears, a new cell separation mechanism must be established to maintain the asymmetric compartments, with N-cadherin taking on this role.
Looping patterns of the midgut are consistent between individuals but can vary across species (Savin et al., 2011). Although differences in growth rates between the gut tube and the DM have been identified as the main contributors to distinct gut looping patterns (Savin et al., 2011), modifications to the kinetics of asymmetry in the DM may also influence these looping patterns, potentially adapting to dietary needs and environmental niches. The presence, permeability, and degradation timing of the midline basement membrane may offer evolution an additional mechanism to fine-tune looping patterns across species. Our findings indicate that this basement membrane is transiently present in the DM of veiled chameleon embryos from just after the 7-somite stage to the 29-somite stage (Figure 3figure supplement 4; Diaz et al., 2019), suggesting conservation of this structure in reptiles and birds, accompanied by intriguing variations in degradation timing across species. In mammals, earlier studies have detected midline laminin deposition in the mouse gut situated between the separating notochord and endoderm, further suggesting the presence of a conserved midline basement membrane in amniotes (Li et al., 2007; Hajduk et al., 2012).
In summary, we have identified a novel midline barrier within the gut mesentery that consists of an atypical double basement membrane. This barrier serves as a critical boundary between the left and right sides, limiting the movement of diffusible signals and cells at a stage when Lefty1 expression is no longer present at the midline. The DM midline offers an exciting opportunity to explore fundamental mechanisms surrounding basement membrane formation and degradation during vertebrate embryonic development, with potential implications for cancer metastasis research. We propose that this midline represents a distinct strategy for effectively separating left and right signals and cells, which is essential for establishing and maintaining LR asymmetry crucial for healthy gut development.