Revolutionary Insights into Olfactory Sensory Neurons and Their Role in Spinal Cord Injury Recovery
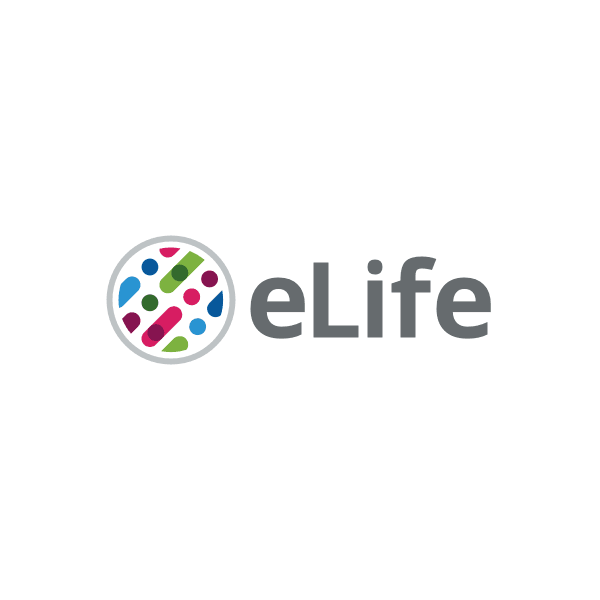
Recent advancements in the study of olfactory sensory neurons (OSNs) have unveiled their exceptional regenerative abilities, particularly in the context of spinal cord injuries. OSNs are distinct in that they engage in neurogenesis throughout an individual's life, a process occurring within the adult olfactory epithelium. This phenomenon was first documented in pioneering research by Graziadei and Monti Graziadei back in 1985, marking a significant milestone in our understanding of neuronal regeneration.
OSNs communicate with specialized glial cells known as olfactory ensheathing cells (OECs), a relationship that was notably highlighted in the work of Doucette in 1990. OECs play a crucial role in aiding the regenerative process of the nervous system, as they offer essential outgrowth and guidance factors. These factors are vital for the axons of OSNs to correctly navigate towards their designated glomerular targets within the brain. The regenerative properties of OECs become particularly significant when addressing neural injuries. Scientific studies have indicated that OECs have the potential to promote axonal regrowth even after severe spinal cord injuries. This potential has been demonstrated across various animal models, including rodents and dogs, as well as in several human studies (Granger et al., 2012; Khankan et al., 2016; Ramn-Cueto et al., 2000; Tabakow et al., 2014; Takeoka et al., 2011; Thornton et al., 2018).
However, the journey toward neural recovery is not without its obstacles. The environment at the site of spinal cord lesions is often inhibitory, featuring reactive glial scars and infiltrating immune cells that significantly hinder the regenerative potential of adult spinal cord neurons (Burda and Sofroniew, 2014; Wanner et al., 2013). The lesion core, primarily composed of non-neural tissue, creates formidable barriers to axonal outgrowth due to the presence of chondroitin sulfate proteoglycans, compounds known to obstruct regeneration effectively (Cregg et al., 2014). In an interesting turn, studies involving the transplantation of OECs following severe spinal cord transections have yielded promising results. These studies suggest that OECs can envelop and support axon bundles as they regenerate, and in certain instances, even extend beyond the injury site (Khankan et al., 2016; Ramn-Cueto et al., 2000; Takeoka et al., 2011; Thornton et al., 2018).
Beyond their supportive role in axonal growth, OECs display properties akin to phagocytes. They actively clear away degenerating axonal debris and necrotic cells from the injury site (Khankan et al., 2016; Nazareth et al., 2020; Su et al., 2013). Furthermore, OECs help modulate the immune response following injury (Khankan et al., 2016; Vincent et al., 2007) and interact favorably with both the glial scar and the lesion core, in both in vitro and in vivo settings (Lakatos et al., 2003; Lakatos et al., 2000). The transplantation of OECs has emerged as a promising strategy for enhancing the conditions for neural repair after complete spinal cord transection (Khankan et al., 2016; Takeoka et al., 2011; Thornton et al., 2018).
Research has shown that OECs secrete several key molecules that promote axon outgrowth. Among these are Brain-derived neurotrophic factor (BDNF), Nerve growth factor (NGF), and Laminin (Ruitenberg et al., 2003; Runyan and Phelps, 2009; Woodhall et al., 2001). These molecules significantly enhance the interactions between neurites and OECs, especially in environments that typically inhibit growth (Chung et al., 2004; Khankan et al., 2015; Windus et al., 2007). Notably, Khankan et al. (2015) found that neurites grew considerably longer in growth-inhibitory conditions when in close proximity to olfactory bulb-derived OECs (OB-OECs), although the specific molecular mechanisms underlying this phenomenon remain to be fully elucidated.
The outcomes of OEC transplantation studies in spinal cord injury (SCI) models vary widely, largely due to differences in experimental design. Factors such as the source of OECs, the severity of the injury, the age of the host, and the timing of transplantation significantly influence the results. Interestingly, OB-OECs appear to have a greater potential for regeneration compared to lamina propria-derived OECs, with studies indicating that freshly prepared OECs are critical for optimal survival rates (Franssen et al., 2007). In a thorough evaluation of 56 studies focusing on OEC transplantation, 41 reported positive outcomes. These included stimulation of regeneration, beneficial interactions with glial scars, and improved axonal remyelination. Recent meta-analyses have confirmed that OECs significantly enhance locomotor functions in SCI models (Watzlawick et al., 2016; Nakhjavan-Shahraki et al., 2018).
In collaboration with a team of researchers, we have conducted six studies on SCI utilizing an adult rat model that involved complete thoracic spinal cord transection, followed by OB-OEC transplantation (Kubasak et al., 2008; Takeoka et al., 2011; Khankan et al., 2016; Thornton et al., 2018; Dixie, 2019). Findings from five of these studies provided both physiological and anatomical evidence supporting axonal regeneration extending into and, in certain cases, beyond the injury site. For example, in long-term studies performed over 6 to 8 months, Takeoka et al. (2011) reported physiological signs of motor connectivity across the transection in rats that received OEC treatment, whereas control groups that received media did not show such connectivity. By utilizing transcranial electric stimulation to measure motor-evoked potentials (MEPs) in the hindlimb muscles, researchers found that 70% of rats treated with OECs exhibited MEPs at 7 months post-transection.
Interestingly, a subsequent re-transection performed a month later resulted in the complete loss of MEPs in OEC-injected rats, providing compelling physiological evidence of axonal conductivity across the injury site. Furthermore, anatomical assessments revealed a significantly higher number of serotonergic axons crossing the rostral inhibitory scar border in rats treated with OECs compared to those that received fibroblast or media controls (Takeoka et al., 2011; Thornton et al., 2018; Dixie, 2019).
The central question of our latest study revolves around the functional capabilities of OB-OECs in the context of neural repair. We propose that distinct subtypes of OB-OECs exist, and that their specific gene expression profiles and the molecules they secrete are pivotal in orchestrating their reparative functions. To investigate this, we employed single-cell RNA sequencing (scRNA-seq) to characterize these OEC subtypes and unravel the molecular programs that facilitate injury repair. We focused on growth-stimulating secreted molecules and cell adhesion factors that enhance the interactions between OB-OECs and promote neurite and axonal outgrowth. Initially, we compared the gene expression profiles of purified OECs with those of non-selected cells from the panning procedure to determine whether the purification process favored specific OEC subtypes. Our scRNA-seq analysis identified five distinct OEC subtypes, each characterized by unique marker genes, which we further validated through experimental methods. Finally, we examined key extracellular matrix (ECM) molecules secreted by OECs, including Reelin (Reln) and Connective Tissue Growth Factor (Ccn2/Ctgf), which may play significant roles in bolstering axonal outgrowth within the challenging microenvironment of the injury core.